What Are Enzymes?
Bioeconomy
Enzymes
Technology
December 19, 2024
1min
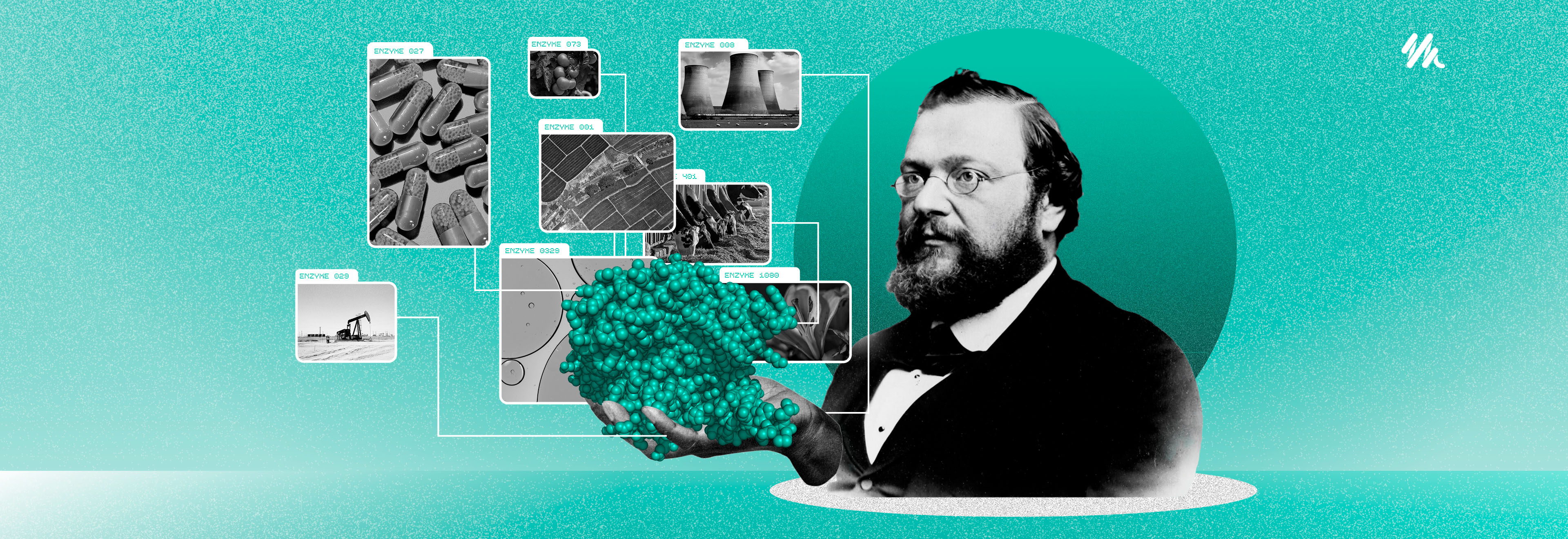
All You Need To Know About Nature's Catalysts Driving Life And Industry
The term enzyme was first coined in 1877 by Wilhelm Friedrich Kühne, a professor of physiology at the University of Heidelberg, derived from a Greek word meaning “to leaven”. But the properties of enzymes were known even earlier, with the first enzyme being discovered by French chemist Anselme Payen in 1833, who first described diastase. This enzyme was later verified as a biological catalyst that could break down starch by Swedish scientist Jöns Jacob Berzelius in 1835, and eventually renamed to its better known term, amylase.
Enzymes are specialized proteins that help to speed up chemical reactions. The vast majority of enzymes are produced by living organisms to assist in all the biochemical functions required to keep them alive. Enzymes are involved in the production and storage of energy, replication and translation of genetic information, construction of cellular architecture, transmission of signals, among other complex biological processes. All of these chemical processes can occur in a narrow range of temperatures and environmental conditions found in living beings. This makes enzymes unique in their ability to produce, modify, and break down chemicals and materials relatively quickly near or above room temperature, but well below the boiling point of water.
(There do exist enzymes found in highly heat-tolerant microorganisms living hot springs known as extremophiles can function above 100 degrees Celsius. But even these enzymes break down above 110 degrees Celsius.)
The simplest example of an enzyme is the one most commonly found in human saliva, amylase. During chewing, amylase is released along with other components in saliva to break down starch. It is specially designed to cleave the chemical linkages between starch, allowing the body to digest smaller starch fragments into sugars that can be absorbed by the stomach. Amylase can cleave starch within minutes while operating at body temperature. Similar chemical processes would require very low pH and boiling temperatures.
Like other proteins, enzymes are made up of amino acids that are encoded in the genome of an organism. Each enzyme is coded in a gene sequence, which must first be transcribed and translated into a functional protein by the organism’s biological machinery before the enzymes can function. They can range in size from less than 100 to more than 2,000 amino acid residues.
These enzyme-coding gene sequences can be inserted into microorganisms, such as yeast or bacteria. The genetically modified microorganisms can be grown in bioreactors to coax them to produce industrial volumes of enzymes for various commercial applications. Enzymes can also be modified by selectively changing the gene sequence from which its produced, which creates a substitution with an amino acid with different characteristics. As we better understand the way enzymes work, these genetic modifications can lead to improved enzyme performance, especially in more challenging chemical environments for native enzymes.
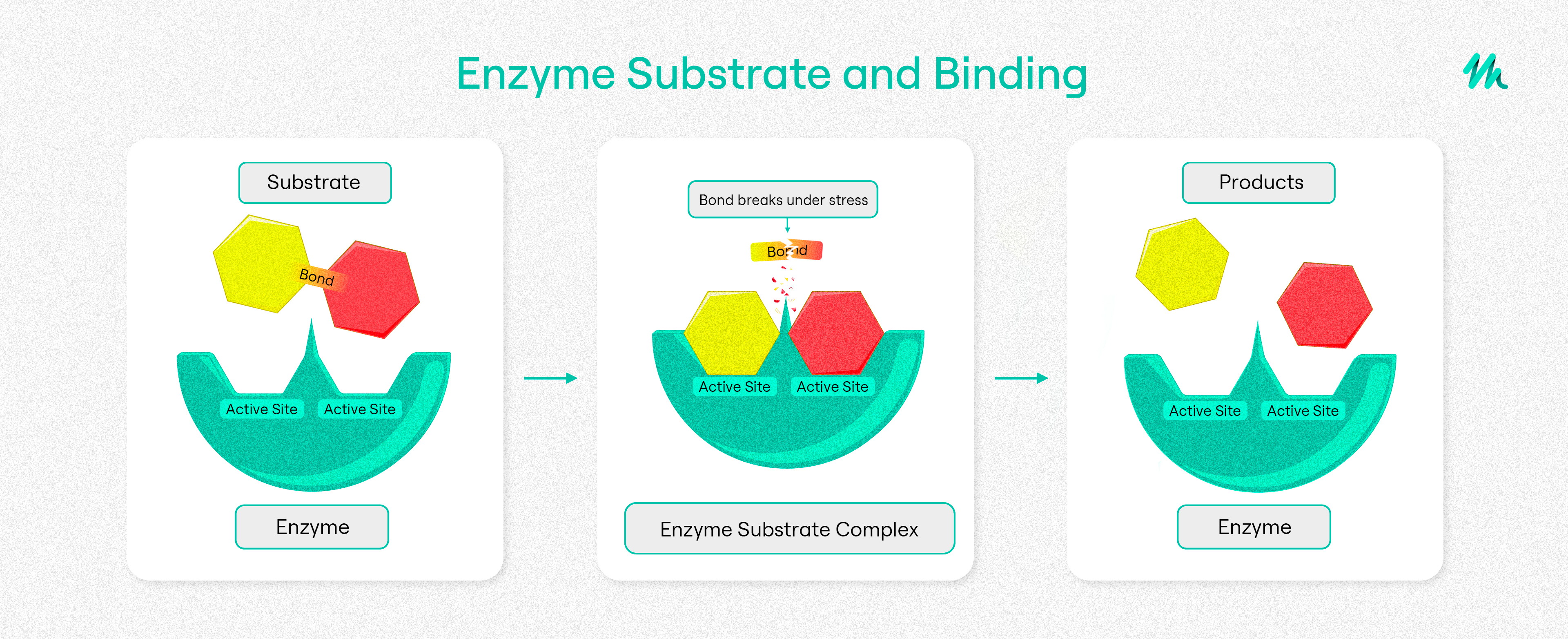
How Do Enzymes Work?
Over the decades, various theories have been posited on how these molecular machines do their work. One model is that enzymes contain pockets or pores on the interior of the protein where substrates can enter. These pockets create a microenvironment that is very different from the external environment. The microenvironment may have a different pH, water activity, salt concentration, or contain certain molecular groups from the amino acids found in the interior of the enzyme that bind to the substrate or substrates. An enzyme may also contain a transition metal that supports the catalytic activity of the protein.
Regardless, these microenvironments help the substrate undergo a series of chemical and structural changes that transform the compound closer to the product. Exchanges between the amino acid residues with the substrate may make certain intermediates more kinetically likely, leading to the production of a new compound or compounds. Once the enzyme has completed the chemical reaction, the enzyme’s initial structure is restored after the reaction. As a result, enzymes can serve as catalysts, as they are not consumed by chemical reactions and can be reused.
Enzymes in Industry
There are several examples where enzymes play a major role in the industry.
In the food and beverage industry, enzymes are essential for the production of food-grade ingredients. Several enzymes are necessary to produce high-fructose corn syrup, a common low-cost sweetener used in the food industry as a replacement for cane sugar. Corn starch is first broken down into a glucose syrup using a combination of alpha-amylase and glucoamylase. Glucose syrup is then run through a column packed with enzymes, xylose and isomerase, and converted into a mixture of fructose and glucose sugars.
In the consumer packaged goods (CPG) industry, environmentally benign detergents are becoming increasingly more visible as an alternative to conventional phosphate-based detergents. These detergents take advantage of enzymes that can break down many of the components found in clothing stains and residues. The major class of enzymes found in enzyme-based detergents is subtilisin, which are capable of rapidly degrading protein-based stains (i.e. blood stains) during washing cycles. Other enzymes are commonly included, such as lipases, which break down fats, and cellulases, which smooths cellulose-based textiles.
Enzymes are also critical for the production of pharmaceuticals. One example is the enzyme penicillin amidase, which catalyzes a key reaction in the production of the antibiotic derivatives of penicillin. Due to the rising threat of antibiotic resistance to penicillin in the 1960s, other antibiotics compounds similar to penicillin were needed. Penicillin could be chemically converted into these other derivatives, but the processes were too costly and time consuming. Penicillin amidase was found to be able to accelerate these steps, which led to breakthroughs in antibiotic manufacturing.
Benefits of Using Enzymes in Manufacturing
Most manufacturing processes require harsh conditions to ensure chemical reactions can proceed to completion, with various combinations of mineral acids, caustic bases, solvents, heavy metal catalysts, and relatively high temperatures. These reaction conditions require workup processes to recover reagents and prevent their release into the surrounding environment. These conditions can also yield side products that may reduce the yield efficiencies of chemical processes or require additional steps to remove contaminants from the final product. Highly specialized processing equipment made up of chemically-resistant alloys are required to contain these reaction systems, which can add additional capital costs to manufacturing operations.
In contrast, enzymes can efficiency achieve high-yield product formation at relatively benign temperatures, where most enzymes are able to operate between 20 to 60 degrees Celsius. This limits the levels of side products formed and reduces the energy requirement to achieve a chemical transformation. Some chemical processes cannot be done without the help of enzymes at all or are completely uneconomical to achieve using only chemical processes alone. Food-grade and cosmetic-grade ingredients for the food and cosmetics industry can be produced at low cost using enzymes without concern of chemical contamination. And processing equipment does not need to be composed of the more costly alloys mentioned above.
Another benefit of enzymes is that they are considered environmentally friendly. When they are released into the environment, they rapidly degrade because they are made up of proteins. That means microorganisms can easily break down the enzymes, leaving no trace after the manufacturing process. They do not require harsh solvents or chemical environments to work as well, with the majority of enzymes functioning optimally in solutions of water, salts, and sugars.
Challenges to Using Enzymes in Manufacturing
Despite the many benefits of using enzymes in manufacturing, they also pose many significant challenges to manufacturers.
For starters, due to the tight environmental conditions required to keep enzymes functioning, enzymes can easily be deactivated by changes in pH, salt concentration, solvent, temperature, light, or even atmosphere. Most enzymes are not able to function in organic solvents commonly used in chemical manufacturing, such as acetone, ethanol, or hexane. Certain chemical manufacturing processes may produce products that also deactivate the enzymes, or reduces their activity over time. Some enzymes require continuous flow of substrate and product, otherwise high concentrations of these compounds could poison the enzyme. As such, complex flow operations are necessary to achieve optimal efficiency.
Enzymes are also costly to produce. The fermentation process to grow and maintain the genetically modified microorganisms requires optimization, constant monitoring, and tight environmental conditions. These bioreactors can experience contamination from external microbial species, or the microorganisms themselves can mutate and yield lower levels of enzyme production over time. Once produced, enzymes need to be properly concentrated, packaged, and kept in optimal conditions during transport, storage, and distribution, all which can add additional costs to the enzyme manufacturing process.
And while enzymes can be recycled, it is still quite technically challenging to separate enzymes from a product or reaction mixture in a usable form. Therefore, it's important to optimize the enzyme properties and the processes to harness enzymes in industrial production.

Designing Better Enzymes
How can we improve enzymes so that they can be better designed to address production challenges?
Nowadays, it’s critical to develop enzymes that can survive and thrive in the hazardous environments needed to produce modern chemicals and materials efficiently. This means designing enzymes that can survive higher temperatures, solvent systems beyond water, high salt conditions, and in the presence of oxygen.
One method for improving enzyme efficiency takes advantage of the fact that enzymes, like other proteins, can be attached to support materials. A process known as immobilization is used to chemically link enzymes to physical supports such as beads or resins. Such immobilized enzymes can be then added to the enzymatic processes for production, separated and recycled for further batches of reactions. Or these beads can also be placed in a column, and the chemical substrate of the enzyme can be diffused through the column. In either case, this improves the recyclability and cost efficiency of the enzymes.
Additionally, new protein design strategies have been developed over the years as advances in synthetic biology have made it easier for genetic and protein engineers to fabricate protein-based machinery. Using the strategy known as directed evolution, a large library of enzyme variants with random or semi-random mutations in their gene sequences are screened to identify the variants carrying more desirable traits. This process is often performed in multiple iterations to increase the chance of finding a better performing enzyme variant. The result is highly engineered enzymes that are capable of outperforming their predecessors in non-natural environments.
An example is the design of the detergent enzymes, subtilisins. Native enzymes are unable to function at the high temperatures and pH conditions found in a normal washing machine. However, through the directed evolution of these enzymes, companies are now able to manufacture engineered versions of these enzymes that are capable of performing in these harsh conditions. Dr. Frances Arnold, a professor of chemical engineering at Caltech, won the Nobel Prize in Chemistry in 2018 for her decades of pioneering work on the subject of directed evolution in enzymes.
The directed evolution strategy has even led to the design of enzymes that can operate in high concentrations of organic solvents, a property not found in nature. Indeed, the methodology has greatly expanded the use of enzymes into chemical reactions and environments that would normally limit their utility.
How We Screen Enzymes
At Allozymes, we use our proprietary microfluidics platform to screen millions of enzyme candidates each day, allowing us to design and optimize enzymes faster than ever before. Our system improves the speed and probability of success that we land successful hits. Our platform also allows us to rapidly prototype, develop, and scale custom enzymes for your specific processes. That means a higher return on value in your manufacturing processes through greater yields, higher efficiencies, and less waste.
For more information, please visit Allozymes’ website and also follow Allozymes on LinkedIn and X.